Socioecological Carbon Production in Managed Agricultural-Forest Landscapes (iSEC)
Exploring the new concept of social C flux and its effect on the carbon cycle in managed agriculture and forest landscapes.
Project Overview
Land use, land cover changes, and ecosystem-specific management practices are increasingly recognized for their roles in mediating the climatic effects on ecosystem structure and function. As demonstrated by some scholars, human activities can influence C fluxes and storage far more than climatic changes (IPCC 2014).
Our understanding and forecasting of ecosystem C fluxes cannot rely solely on conventional biophysical regulations at any scale, from the local ecosystem to the globe. We must quantify the magnitude of the C fluxes from managed ecosystems and landscapes over the lifetime of the C cycle and deduct the various energy inputs during management from the amount of C sequestered by an ecosystem (West & Marland 2003). For example, conventional crop management often includes tillage, fertilization, irrigation, applications of pesticides and herbicides, harvesting, transportation to the market, land conversion, etc. All of these activities require a CO2-equivalent (CO2eq) amount of energy (“social C flux”) to offset the actual amount of C sequestered by the ecosystems and landscapes. A complete life cycle assessment (LCA) is needed to account for the actual sequestration strength at different spatial and temporal scales.
Our overall objective is to quantify the landscape-scale C fluxes at annual scale of both managed agricultural-forest landscapes and people, using the Kalamazoo watershed in southwestern Michigan as our testbed.
Questions to be answered
- (1) What are the quantitative contributions of land cover change, specific management practices, and climate changes (means and extremes) to the social and physical C fluxes of managed ecosystems and landscapes?
- (2) What are the spatial and temporal changes of their contributions in managed agricultural-forest landscapes?
- (3) How will future land use changes (including alternative management practices) impact C sequestration in an upper, mid-latitude managed ecosystem?
Acknowledgements
Funded by NASA Carbon Cycle & Ecosystems (CC&E) Download project summary
Recent Activity
- April 2019: Gabriela Shirkey (PhD student) is awarded the NSF GRFP starting fall 2019
- March 2019: PhD students Cheyenne Lei and Gabriela Shirkey awarded College of Social Science Research Endowment
- Fall 2018: Pietro Scuisco (PhD student) wins travel grant to USA IALE
- Summer 2018: Social Carbon team distributed 500 paper surveys with online access and traveled to 25 homes for in-person interviews
- Fall 2018: David Reed (Postdoctoral Research Associate), Gabriela Shirkey (PhD student), and Jeralyn Poe (Undergraduate) attend AGU 2018 to present posters and host session "Land Management in the Earth System: Measurements and Models I"
- March 27-30, 2017: NACP & AmeriFlux PI Meeting
- July 10, 2017: First classification complete!
-
Changes in land cover type across the Kalamazoo Watershed (Landsat 30m resolution data) demonstrate the increasing rates of urbanization and from 1996 to 2011. Credit: Rong Zhang - May-July 2017: Five flux towers can be found across the Kalamazoo Watershed representing urban, marsh, forest, and agricultural land.
- March 20, 2017: Annual Legislative Breakfast hosted by the Kalamazoo Environmental Council.
Conceptual Framework and Hypothesis
Our overarching hypothesis is that social C flux is more responsible than physical C flux for the dynamics, and especially the uncertainty, of the cumulative CO2eq production of these intensively-managed landscapes. However, their proportions vary significantly among the landscapes and over history because of the great variations in land conversions, land use practices, climatic changes and extremes in the watershed.
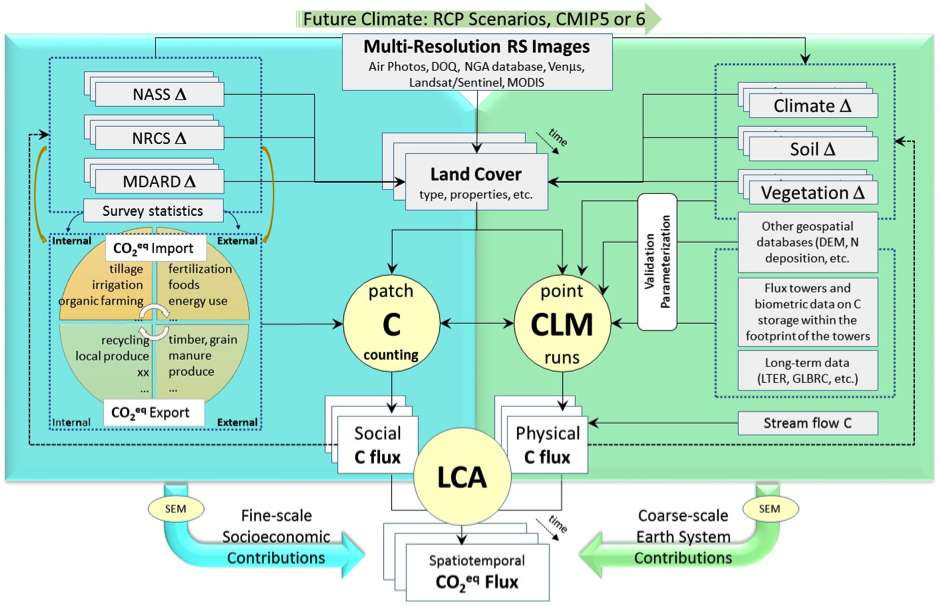
Figure 1. (above) Proposed research components and their linkages for process-based predictions of the spatiotemporal changes in CO2eq production that will be quantified by estimating “social C flux”, and “physical C flux” at contrasting landscapes (i.e., different land cover compositions) within the Kalamazoo Watershed as well as the entire watershed (Fig. 2, below).
Life cycle assessment (LCA) will be employed for major patch types to quantify the C production at different temporal scales. The statistical downscaling modeling will be used to predict future local climate from Representative Concentration Pathways scenarios. Bayesian structural equation models (SEM) will be constructed to explore the contributions of climate change and human activities.
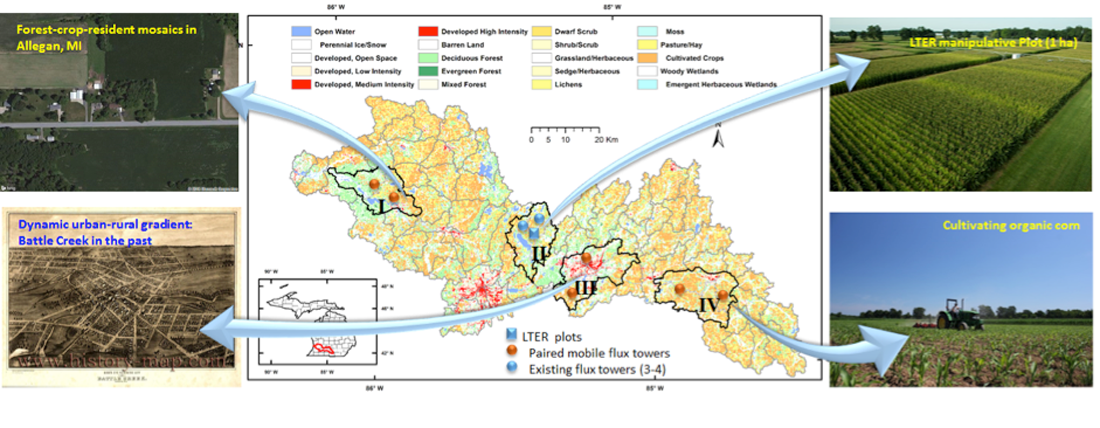
Figure 2. (above) Current land cover of the Kalamazoo Watershed (NLCD), which includes 127 sub-watersheds (USGS). The entire watershed will be examined for the changes of CO2eq during a 40-year period (1978–2018) using Landsat/Sentinel with the climate and human activities following our working framework (Fig. 1), while four contrasting landscapes will be quantified with high-resolution RS data and historical records and survey statistics over an 80-year period (1938–2018).
Research Tasks
Task 1: Dynamics of Physical C Fluxes
Quantify the changes of the physical C flux on an annual scale, which will be converted to CO2eq, by integrating: (1) remotely-sensed land cover type and other surface properties; (2) geospatial records of climate, vegetation, soil, and management practices for model parameterization; (3) direct measurements of net ecosystem exchange of CO2 using EC flux towers for model validation; and (4) a customized ecosystem model (i.e. CLM).
Task 2: Dynamics of Social C Fluxes
Estimate the social C fluxes of major management practices for different land cover types by classifying historical land cover, identifying land ownership, and by surveying historical management practices of individual land-owners (parcel scale). Back-of-the-envelope calculations will be applied to scale up the CO2eq fluxes to the landscapes and the watershed.
Task 3: The dynamics and the regulations of CO2eq in time and space
Diagnose the mechanistic/empirical causal relationships based on biophysical models and SEM, and to quantify the ecosystem, landscape, and watershed C fluxes at multiple temporal scales and under alternative management/climate scenarios.
Open Data Resources
In alliance with NASA, our researchers openly share their data with the broader community. The latest information and resources from our project can be found here for exploration and education.
Data use policy
Use of this data is for non-profit, personal, or educational use only. To publish this data or apply it in other for-profit endeavours, please contact Dr. Jiquan Chen for information. For help interpreting the data, contact the author listed. Information provided here does not conflict with our committment to confidentiality or expose personal information from survey participants.
View/Download Data:
- Eddy-Covariance Flux Data
- Geospatial and Remote Sensing Data
- Socio-Economic Carbon Data
- Community Land Model Data
- Background Information Database
References
- Abraha, M., Gelfand, I., Hamilton, S. K., Shao, C., Su, Y.-J., Robertson, G. P., & Chen, J. (2016). Ecosystem Water-Use Efficiency of Annual Corn and Perennial Grasslands: Contributions from Land-Use History and Species Composition. Ecosystems, 1-12.
- Amiro, B.D., A.G. Barr, J.G. Barr, T.A. Black, R. Bracho, M. Brown, J. Chen, et al. (2010). Ecosystem carbon dioxide fluxes after disturbance in forests of North America. Journal of Geophysical Research - Biogeosciences 115(G4), G00K02. DOI: 10.1029/2010JG001390
- Arhonditsis, G. B., Stow, C. A., Steinberg, L. J., Kenney, M. A., Lathrop, R. C., McBride, S. J., & Reckhow, K. H. (2006).Exploring ecological patterns with structural equation modeling and Bayesian analysis. Ecological Modelling, 192(3–4), 385-409.
- Bass, D.G. (2009). Inferring dissolved phosphorus cycling in a TMDL watershed using biogeochemistry and mixed linear models. Ph.D. Thesis, Michigan State University, East Lansing, Michigan.
- Champman, K. A., and Brewer, R. (2008). Prairie and savanna in southern Lower Michigan: history, classification, ecology. Mich. Bot., 47:1-48.
- Chen, J., Brosofske, D. K., Noormets, A., Crow, R. T., Bresee, K. M., Le Moine, et al. (2004). A Working Framework for Quantifying Carbon Sequestration in Disturbed Land Mosaics. Environmental Management, 33(1), S210-S221.
- Chen, J., Davis, K. J., & Meyers, T. P. (2008). Ecosystem–atmosphere carbon and water cycling in the upper Great Lakes Region. Agricultural and Forest Meteorology, 148(2), 155-157.
- Chen, J., John, R., Shao, C., Fan, Y., Zhang, Y., Amarjargal, Dong, G. (2015). Policy shifts influence the functional changes of the CNH systems on the Mongolian plateau. Environmental Research Letters, 10(8), 085003
- Chu, H., Chen, J., Gottgens, J. F., Desai, A. R., Ouyang, Z., & Qian, S. S. (2016). Response and biophysical regulation of carbon dioxide fluxes to climate variability and anomaly in contrasting ecosystems in northwestern Ohio, USA. Agricultural and Forest Meteorology, 220, 50-68.
- Cressie, N. & Wikle., C. (2011). Statistics for spatio-temporal data. Wiley press.
- Drewniak, B., Song, J., Prell, J., Kotamarthi, V. R., & Jacob, R. (2013). Modeling agriculture in the Community Land Model. Geosci. Model Dev., 6(2), 495-515.
- Estoque, R. C., & Murayama, Y. (2015). Classification and change detection of built-up lands from Landsat-7 ETM+ and Landsat-8 OLI/TIRS imageries: A comparative assessment of various spectral indices. Ecological Indicators, 56, 205-217.
- Euskirchen, E. S., Chen, J., Li, H., Gustafson, E. J., & Crow, T. R. (2002). Modeling landscape net ecosystem productivity (LandNEP) under alternative management regimes. Ecological Modelling, 154(1–2), 75-91.
- Fan, Y., Wu, R., Chen, J., & Apul, D. (2015). A Review of Social Life Cycle Assessment Methodologies. In S. S. Muthu (Ed.), Social Life Cycle Assessment: An Insight (pp. 1-23). Singapore: Springer Singapore.
- Fongers, D. (2008). Kalamazoo River Watershed Hydrologic Study. Retrieved from http://kalamazooriver.org/wp-content/uploads/2012/12/DEQ-Kalamazoo-River-Hydrologic-Study-2008.pdf
- Gelfand, A. E., & Smith, A. F. M. (1990). Sampling-Based Approaches to Calculating Marginal Densities. Journal of the American Statistical Association, 85(410), 398-409.
- Gelfand, I., Zenone, T., Jasrotia, P., Chen, J., Hamilton, S. K., & Robertson, G. P. (2011). Carbon debt of Conservation Reserve Program (CRP) grasslands converted to bioenergy production. Proceedings of the National Academy of Sciences, 108(33), 13864-13869.
- Grace, J. B., Anderson, T. M., Olff, H., & Scheiner, S. M. (2010). On the specification of structural equation models for ecological systems. Ecological Monographs, 80(1), 67-87.
- Hamilton, S. K., Hussain, M. Z., Lowrie, C., Basso, B., & Robertson, G. P. (in review) Evapotranspiration response to land cover and climate change in a Midwest U.S. watershed. Scientific Reports.
- Hurrell, J. W., Holland, M. M., Gent, P. R., Ghan, S., Kay, J. E., Kushner, et al. (2013). The Community Earth System Model: A Framework for Collaborative Research. Bulletin of the American Meteorological Society, 94(9), 1339-1360.
- Hurtt, G. C., Chini, L. P., Frolking, S., Betts, R. A., Feddema, J., Fischer, G. et al. (2011). Harmonization of land-use scenarios for the period 1500–2100: 600 years of global gridded annual land-use transitions, wood harvest, and resulting secondary lands. Climatic Change, 109(1), 117-161.
- IPCC. (2014). Climate Change 2014: Synthesis Report. Contribution of Working Groups I, II and III to the Fifth Assessment Report of the Intergovernmental Panel on Climate Change [Core Writing Team, R.K. Pachauri and L.A. Meyer (Eds.)]. IPCC, Geneva, Switzerland, 151 pp.
- Koellner, T. (2002). Land use in product life cycles and its consequences for ecosystem quality. The International Journal of Life Cycle Assessment, 7(2), 130-130.
- Koellner, T., de Baan, L., Beck, T., Brandão, M., Civit, B., Margni, M., et al. (2013). UNEP-SETAC guideline on global land use impact assessment on biodiversity and ecosystem services in LCA. The International Journal of Life Cycle Assessment, 18(6), 1188-1202.
- Koellner, T., & Geyer, R. (2013). Global land use impact assessment on biodiversity and ecosystem services in LCA. The International Journal of Life Cycle Assessment, 18(6), 1185-1187.
- Koellner, T., & Scholz, R. W. (2006). Assessment of land use impacts on the natural environment. The International Journal of Life Cycle Assessment, 13(1), 32-48. doi:10.1065/lca2006.12.292.2
- Lal, R. (2011). Reducing emissions and sequestering carbon in agroecosystems. Food Policy, 36, S33-S39.
- Levasseur, A., P. Lesage, M. Margni, L. Deschênes, & R. Samson. (2009). How dynamic LCA can bring consistency in assessing global warming mitigation scenarios: Paper presented at Life Cycle Assessment IX: toward the global life cycle economy. Boston, MA
- Levis, S., Badger, A., Drewniak, B., Nevison, C., & Ren, X. (2016). CLMcrop yields and water requirements: avoided impacts by choosing RCP 4.5 over 8.5. Climatic Change, 1-15.
- Levis, S., Hartman, M. D., & Bonan, G. B. (2014). The Community Land Model underestimates land-use CO2 emissions by neglecting soil disturbance from cultivation. Geosci. Model Dev., 7(2), 613-620.
- Mao, J., Ricciuto, D. M., Thornton, P. E., Warren, J. M., King, A. W., Shi, X., et al. (2016). Evaluating the Community Land Model in a pine stand with shading manipulations and 13CO2 labeling. Biogeosciences, 13(3), 641-657.
- Marland, G., West, T. O., Schlamadinger, B., & Canella, L. (2003). Managing soil organic carbon in agriculture: the net effect on greenhouse gas emissions. Tellus B, 55(2), 613-621.
- Millar, N., Robertson, G.P., Grace, P.R., Gehl, R.J. and Hoben, J.P. (2010). Nitrogen fertilizer management for nitrous oxide (N2O) mitigation in intensive corn (Maize) production: an emissions reduction protocol for US Midwest agriculture. Mitig Adapt Strateg Glob Change, 15,185–204.
- Milà i Canals, L., Bauer, C., Depestele, J., Dubreuil, A., Freiermuth Knuchel, R., Gaillard, et al. (2007). Key elements in a framework for land use impact assessment within LCA (11 pp). The International Journal of Life Cycle Assessment, 12(1), 5-15.
- Mladenoff, D. J. (2004). LANDIS and forest landscape models. Ecological Modelling, 180(1), 7-19.
- Noriega, A. E., & de Alba, E. (2001). Stationarity and structural breaks — evidence from classical and Bayesian approaches. Economic Modelling, 18(4), 503-524.
- Oleson, K. W., Lawrence, D. M., Bonan, G. B., Drewniak, B., Huang, M., Koven, et al. (2013). Technical Description of version 4.5 of the Community Land Model (CLM). Boulder, CO: National Center for Atmospheric Research. Retrieved from http://n2t.net/ark:/85065/d74f1q4q
- Papale, D., Black, T. A., Carvalhais, N., Cescatti, A., Chen, J., Jung, et al. (2015). Effect of spatial sampling from European flux towers for estimating carbon and water fluxes with artificial neural networks. Journal of Geophysical Research: Biogeosciences, 120(10), 1941-1957.
- Poursanidis, D., Chrysoulakis, N., & Mitraka, Z. (2015). Landsat 8 vs. Landsat 5: A comparison based on urban and peri-urban land cover mapping. International Journal of Applied Earth Observation and Geoinformation, 35, Part B, 259-269.
- Reap, J., Roman, F., Duncan, S., & Bras, B. (2008). A survey of unresolved problems in life cycle assessment. The International Journal of Life Cycle Assessment, 13(5), 374-388.
- Robertson, G. P., Paul, E. A., & Harwood, R. R. (2000). Greenhouse Gases in Intensive Agriculture: Contributions of Individual Gases to the Radiative Forcing of the Atmosphere. Science, 289(5486), 1922-1925.
- Saad, R., Margni, M., Koellner, T., Wittstock, B., & Deschênes, L. (2011). Assessment of land use impacts on soil ecological functions: development of spatially differentiated characterization factors within a Canadian context. The International Journal of Life Cycle Assessment, 16(3), 198-211.
- Schaefer, K., Schwalm, C. R., Williams, C., Arain, M. A., Barr, A., Chen, et al. (2012). A model-data comparison of gross primary productivity: Results from the North American Carbon Program site synthesis. Journal of Geophysical Research: Biogeosciences, 117(G3), n/a-n/a. doi:10.1029/2012JG001960
- Schaetzl, R. J., Darden, J. T., & Brandt, D. S. (2009). Michigan geography and geology. New York: Custom Publishing.
- Shao, C., Chen, J., & Li, L. (2013). Grazing alters the biophysical regulation of carbon fluxes in a desert steppe. Environmental Research Letters, 8(2), 025012.
- Smith, P., Andrén, O., Karlsson, T., Perälä, P., Regina, K., Rounsevell, M., & Van Wesemael, B. (2005). Carbon sequestration potential in European croplands has been overestimated. Global Change Biology, 11(12), 2153-2163.
- The United Nations, Framework Convention on Climate Change. (2015). Adoption of the Paris Agreement. Retrieved from: https://unfccc.int/resource/docs/2015/cop21/eng/l09r01.pdf
- West, T. O., & Marland, G. (2003). Net carbon flux from agriculture: Carbon emissions, carbon sequestration, crop yield, and land-use change. Biogeochemistry, 63(1), 73-83.
- West, T. O., & Post, W. M. (2002). Soil Organic Carbon Sequestration by Tillage and Crop Rotation: A Global Data Analysis. Soil Science Society of America Journal, 66, 1930-1946.
- Wiedmann, T. and Minx, J. (2008). A definition of ‘carbon footprint’. Ecological Economics Research Trends, 1, 1-11.
- Wu, R., Yang, D., & Chen, J. (2014). Social Life Cycle Assessment Revisited. Sustainability, 6(7), 4200.
- Xiao, J., Chen, J., Davis, K. J., & Reichstein, M. (2012). Advances in upscaling of eddy covariance measurements of carbon and water fluxes. Journal of Geophysical Research: Biogeosciences, 117(G1), n/a-n/a. doi:10.1029/2011JG001889
- Xie, J., Chen, J., Sun, G., Zha, T., Yang, B., Chu, H., et al. (2016). Ten-year variability in ecosystem water use efficiency in an oak-dominated temperate forest under a warming climate. Agricultural and Forest Meteorology, 218–219, 209-217.
- Zenone, T., Chen, J., Deal, M. W., Wilske, B., Jasrotia, P., Xu, et al. (2011). CO2 fluxes of transitional bioenergy crops: effect of land conversion during the first year of cultivation. GCB Bioenergy, 3(5), 401-412.
- Zenone, T., Gelfand, I., Chen, J., Hamilton, S. K., & Robertson, G. P. (2013). From set-aside grassland to annual and perennial cellulosic biofuel crops: Effects of land use change on carbon balance. Agricultural and Forest Meteorology, 182–183, 1-12.
- Zimmerman, P.R., Price, M, Peng, C., Capehart, W.J., Updegraff, K., Kozak, P., et al. (2005). C-lock (patent pending): a system for estimating and certifying carbon emission reduction credits for the sequestration of soil carbon on agricultural land. Mitigation and Adaptation Strategies for Global Change, 10, 307–331.
Products and Presentations
Publications
- Dahlin, K. M., Akanga, D., Lombardozzi, D. L., Reed, D. E., Shirkey, G., Lei, C., Abraha, M., Chen, J. 2020. Challenging a Global Land Surface Model in a Local Socio-Environmental System. Land. 9(10), 398. DOI: 10.3390/land9100398
- Reed, D.E., Chen, J., Abraha, M., Robertson, G.P. and Dahlin, K.M., 2020. The Shifting Role of mRUE for Regulating Ecosystem Production. Ecosystems, 23(2), pp.359-369.
- Chen, J., Sciusco, P., Ouyang, Z., Zhang, R., Henebry, G.M., John, R. and Roy, D.P., 2019. Linear downscaling from MODIS to Landsat: connecting landscape composition with ecosystem functions. Landscape Ecology, 34(12), pp.2917-2934.
- Sciusco, P., Chen, J., Abraha, M., Lei, C., Robertson, G.P., Lafortezza, R., Shirkey, G., Ouyang, Z., Zhang, R. and John, R., 2020. Spatiotemporal variations of albedo in managed agricultural landscapes: inferences to global warming impacts (GWI). Landscape Ecology, 35(6), pp.1385-1402.
- Poe, J., Reed, D.E., Abraha, M., Chen, J., Dahlin, K.M. and Desai, A.R., 2020. Geospatial coherence of surface-atmosphere fluxes in the upper Great Lakes region. Agricultural and Forest Meteorology, 295, p.108188.
- Reed, D., J. M Poe, M. Abraha, and J. Chen. Geospatial Land-Surface Fluxes: Modeled spatial fluxes from paired sites using neural networks. Agriculture and Forest Meteorology (to be submitted; abstract submitted to AGU 2019)
- Reed, D., C. Lei, W. Baule, G. Shirkey, J. Chen and K. Czajkowski. Impacts of an urban density gradient on land-surface fluxes. Environmental Research Letters (to be submitted); abstract submitted to AGU 2019)
- Dahlin, K.M., Akanga, D., Lombardozzi, D., Reed, D.E., Shirkey, G., Abraha, M., and J. Chen. Challenging a global land surface model at the watershed scale. JGR: Biogeosciences. (to be submitted; abstract submitted to AGU 2019)
- Shirkey, G., M. Abraha, D. Reed, J. Chen, J. Baker, C. Bernacchi, T. Griffis, T. Meyers, J. Prueger, A. Suyker. Integrating net ecosystem production and socioeconomic factors in corn-soy growing regions of the US. Ecology and Society. (to be submitted)
- Lei, C., M. Abraha, Y.J. Su, and J. Chen. Variability of root production in bioenergy systems using ingrowth cores and eddy covariance. Journal of Plant Ecology (accepted )
Presentations
- Dahlin, K. M., Akanga, D., Lombardozzi, D. L., Reed, D. E., Shirkey, G., Lei, C., Abraha, M., Chen, J. 2020. Challenging a Global Land Surface Model in a Local Socio-Environmental System. North American Carbon Program. January 2021 Highlights.
- Chen, J., P. Sciusco, R. John et al. Carbon and water fluxes at high spatial resolutions through down-scaling. Japan Geoscience Union Meeting, 5/28/2019, Chiba, Japan
- John, R., Chen, J., Sciusco, P., Shirkey, G., John, R., Ouyang, Z., Lei, C., Zhang, R., and Lafortezza, iSEC: Integrated Socioecological Carbon Production in Managed Agricultural-Forest Landscapes: Intra-annual variation of albedo due to land cover/use change: contributions to global warming impacts in agricultural landscapes. NASA LCLUC Science Team Meeting 2019, Rockville, MD, April 9-11, 2019
- Akanga, DO. 2019, Carbon Cycle Change in Kalamazoo River Watershed. NCAR 2019 CLM/CTSM Tutorial Workshop, Boulder, CO February 4-8, 2019 (poster)
- Sciusco P., J. Chen, P. Robertson, Z. Ouyang, L. Cheyenne, J. Ranjeet, Z. Rong, R. Lafortezza. Spatiotemporal variations of albedo due to land use: contributions to global warming impacts in managed agricultural landscapes. US-IALE 2019 Annual Meeting, April 7-11, 2019, Fort Collins, CO. (poster)
- Sciusco P., J. Chen, P. Robertson, Z. Ouyang, L. Cheyenne, J. Ranjeet, Z. Rong, R. Lafortezza. Spatiotemporal variations of albedo due to land use: contributions to global warming impacts in managed agricultural landscapes. US-IALE 2019 Annual Meeting, April 7-11, 2019, Fort Collins, CO. (poster)
- Reed, D., J. Poe, M. Abraha, J. Chen. Fluxes from Across the Street: Using artificial neural networks to model carbon cycling from paired flux sites. AGU Annual Meeting, December 2018, Washington D.C., USA.
- Poe, J., D. Reed, A. Desai, J. Chen, M. Abraha, S. Metzger. Spatial Coherence of Carbon Flux Towers in Midwest Ecosystems. AGU Annual Meeting, December 2018, Washington D.C., USA.
- Reed, D. Estimating landscape level surface flux observations from a single tower. University of Wisconsin-Madison, November 2018, Madison, WI, USA.
- Reed, D. Multiple Resource Use Efficiency (mRUE) In Agriculture Systems. Conference on Agricultural and Forest Meteorology, May 2018, Boise, ID, USA.
- Shirkey, G., P. Sciusco, R. John, D. Reed, K. O'Brien, L. Cooper, J. Chen, K. Dahlin. Integrating historical land cover and land management in Michigan’s Kalamazoo Watershed: a story of carbon flux impact.Great Lakes Bioenergy Research Center Annual Science Meeting Poster Session, May 2018, Lake Geneva, WI, USA.
- Shirkey, G. Implementing an agricultural Life Cycle Assessment (LCA) at the regional scale in southwest Michigan. Global Change and Agroecosystems: Challenges and Opportunities Poster Session, January 2018, East Lansing, MI, USA.
- Chen, J., K. Dahlin, R. John, G. Shirkey, S. R. Wu, P. Robertson, S. Hamilton, L. Cooper, D. Lusch, and A. Karnieli, R. Lafortezza, and G. S. Labini. Socioecological Carbon Production in Managed Agricultural-Forest Landscapes. 2017 Joint NACP & AmeriFlux PI Meeting, March 27-30, 2017, North Bethesda, MD, USA.
- Chen, J., K. Dahlin, R. John, G. Shirkey, S. R. Wu, P. Robertson, S. Hamilton, L. Cooper, D. Lusch, and A. Karnieli, R. Lafortezza, and G. S. Labini. Socioecological Carbon Production in Managed Agricultural-Forest Landscapes. Worldcover 2017 Conference, 14–16 March 2017, Rome, Italy.
- Dahlin, K. Resolution and the Carbon Cycle: Spatial, temporal, and spectral variation in ecosystems. Invited seminar for the Wayne State University Department of Biological Sciences. Nov. 13, 2017.
- Chen, J. Institution in Ecosystem Analysis: A Forgotten Driver. Distinguished Ecologist Lecture Series (DELS), School of Forest Resources and Environmental Science, Michigan Tech University, Oct. 26, 2017.
Team Members
![]() |
Dr. Jiquan Chen | Professor, PI Michigan State University |
jqchen@msu.edu |
![]() |
Dr. Kyla Dahlin | Assistant Professor, Co-PI Michigan State University |
kdahlin@msu.edu |
![]() |
Dr. Ranjeet John | Research Associate, Co-PI Michigan State University |
ranjeetj@msu.edu |
![]() |
Dr. Phil Robertson | Collaborator Kellogg Biological Station (GLBRC) |
robert30@msu.edu |
![]() |
Dr. Steve Hamilton | Collaborator Kellogg Biological Station (LTER) |
hamilton@msu.edu |
![]() |
Dr. David Lusch | Collaborator, RS/GIS Michigan State University |
lusch@msu.edu |
![]() |
Lauren Cooper | Collaborator, Forest Climate Program Michigan State University | ltcooper@msu.edu |
![]() |
Dr. Arnon Karnieli | CollaboratorBen Gurion University, Israel | karnieli@bgu.ac.il |
![]() |
Giovanni Sylos Labini | Collaborator Planetek, Italy | website |
![]() |
Rong Zhang | Collaborator Michigan State University | zhangr38msu.edu |
![]() |
Dr. David Reed | Postdoctoral Research Associate Michigan State University | reeddav2@msu.edu |
![]() |
Gabriela Shirkey | PhD Student Michigan State University | shirkeyg@msu.edu |
![]() |
Ryan Nagelkirk | PhD Student Michigan State University | nagelki4@msu.edu |
![]() |
Kaitlyn O'Brien | Undergraduate Student Michigan State University | obrie227@msu.edu |
![]() |
Connor Crank | Research Technologist Michigan State University | crankcon@msu.edu |
![]() |
Cheyenne Lei | PhD Student Michigan State University | cheyenne@msu.edu |
![]() |
Zutao Ouyang | Postdoctoral Research Associate Michigan State University | |
![]() |
Donald Akanga | PhD Student Michigan State University | akangado@msu.edu |